Our research group focusses on the design of electron relays to bridge redox proteins and electrodes for energy conversion and energy saving schemes. Redox enzymes are highly active and selective catalysts for various reactions and are hence attractive for technological applications. We use synthetic chemistry to build redox interfaces on electrode surface to accommodate the target biomolecules with respect to their properties and intended applications. Our main projects involve biosensing, biophotovoltaics, biofuel cells and bioelectrocatalysis.
Projects
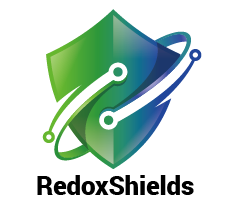
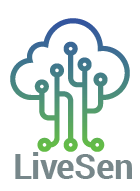
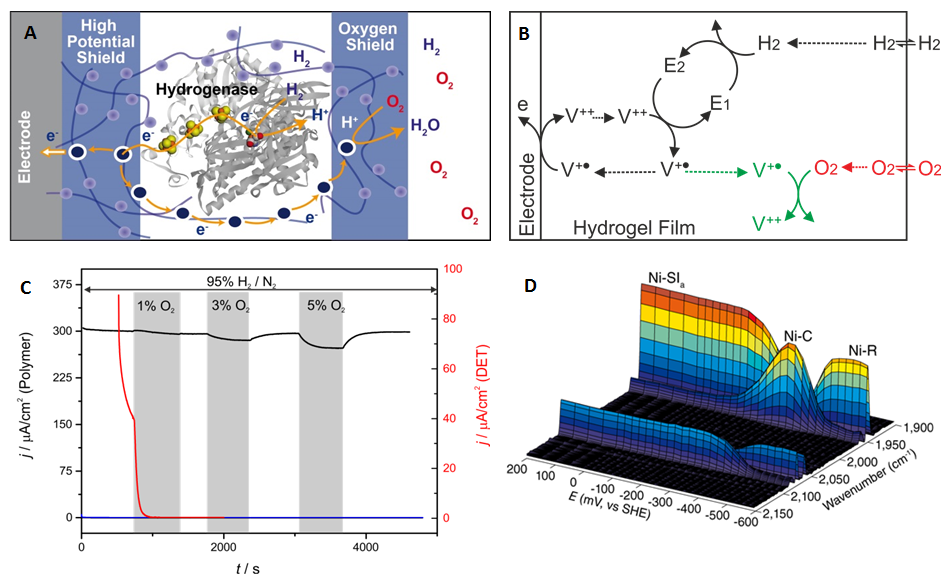
Redox enzymes may play a role as substitute for noble metal in devices for conversion of chemical fuel to electricity. The main issue is the stability of the biocatalysts. We have shown that the electron mediator can be designed to protect the catalyst from various degradation processes to achieve stability over weeks unter constant turn-over conditions for H2 oxidation in biofuel cell1.
We apply our expertise in chemistry and electrochemistry to develop novel strategies to protect sensitive catalysts from the harsh conditions encountered in fuel cells and other technological devices under operation2. To date only materials based on noble metals reached technological applications. In cooperation with W. Schuhmann, O. Rüdiger and W. Lubitz, we designed a redox hydrogel that serves as a protecting matrix for the catalyst3. The concept was successfully demonstrated with a hydrogenase as model for an efficient hydrogen oxidizing catalyst with extreme sensitivity. The hydrogel concept, reported in Nature Chemistry, opens the possibility to integrate a variety of other sensitive biological or artificial catalyst for which the intrinsic stability cannot be further increased. This is a major step toward a novel fuel cell redesign, which may reposition them at the forefront in the race toward global sustainable energy supply.
- Plumeré N., Rüdiger O., Alsheikh Oughli A., Williams R., Vivekananthan J., Pöller S., Schuhmann W., Lubitz W. Nature Chemistry, 2014, 6, 822–827. DOI: 10.1038/nchem.2022
- Alsheikh Oughli A., Conzuelo F., Winkler M., Happe T., Lubitz W., Schuhmann W., Rüdiger O., Plumeré N. Angew. Chem. Int. Ed., 2015, 54 (42), 12329–12333. DOI: 10.1002/anie.201502776
- Fourmond V., Stapf S., Li H., Buesen D., Birrell J., Olaf R.; Lubitz W., Schuhmann W., Plumeré N., Léger C. J. Am. Chem. Soc., 2015, 137, 5494-5505. DOI: 10.1021/jacs.5b01194
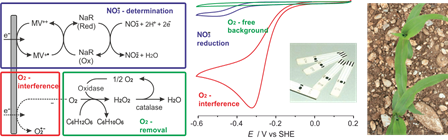
This research topic targets a novel sensing approach for sparing the fossil energy resources massively used in agriculture. The production of nitrogen fertilizer alone consumes up to 5% of the global natural gas production. These fertilizers are applied in excess in the field and lost in the form of nitrates. Our group addresses this issue by developing sensors to determine the nitrate content in plants directly in the field by the farmers themselves to provide the information on the plants’ need to adjust the fertilizer input in real-time1. The democratization of the use of such sensors requires simple procedures for on-site nitrate quantification without sample preparation. To this end a redox enzyme that selectively reduces nitrate2 is contacted via artificial electron relays to single-use electrodes. The main interferences in this sensing concept when brought from the lab to field applications is oxygen from air. To overcome this issue, we develop universal oxygen scavengers that are compatible with biochemical and electrochemical processes. The principal challenge resides in designing an oxygen reduction reaction that results in fully inert products3. Enzymatic oxygen scavengers based on carbohydrate oxidation are most promising and we selected the enzymes catalase and pyranose oxidase to reduce oxygen with glucose to water. Such O2 scavenging systems maintain tiny volumes (down to 20 µL) under anaerobic conditions for hours, even when exposed to ambient air. The conditions of the systems such as pH value or biological activity are not affected by the oxygen reduction product4,5. We demonstrated that the oxygen scavenger system associated to the nitrate biosensing concept allow for fast, simple and reliable nitrate quantification on the field.
The impact of the nitrate biosensing method to spare fossil ressources and to eventually reduce CO2 emission was recently recognized by KlimaExpo.NRW who made it a priority topic to encourage and educate about climate protection6. The method is awaiting validation of a pending patent application7.
- Plumeré N. Nachrichten aus der Chemie 2014, 62(7), 777-779. DOI: 10.1002/nadc.201490261
- Campbell W. H., Henig J., Plumeré N. Bioelectrochemistry, 2013, 93, 46-50. DOI: 10.1016/j.bioelechem.2012.07.002
- Plumeré N. Analytical and Bioanalytical Chemistry, 2013, 405(11), 3731-3738. DOI: 10.1007/s00216-013-6827-z
- Swoboda M., Henig J., Cheng H.-M., Brugger D., Haltrich D., Plumeré N., Schlierf M. ACS Nano, 2012, 6(7), 6364-6369.DOI: 10.1021/nn301895c
- Plumeré N., Henig J. and Campbell W. H. Analytical Chemistry, 2012, 84, 2141-2146. DOI: 10.1021/ac2020883
- Plumeré N.,Nitratsensor: Schluss mit Überdüngung – Feldanalytik in der Landwirtschaft. KlimaExpo.NRW Motor für den Fortschritt
- Plumeré N., Campbell W. H., Campbell E. R., Systems and Methods for Enzymatic Oxygen Removal. US2012211372 (A1) – 2012-08-23
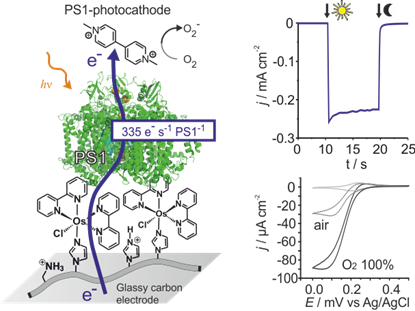
Besides organic and inorganic semiconductors, that have reached practical efficiencies and commercial applications, the utilization of photosynthetic material in artificial device to convert light energy is principally demonstrated1. Currently there is a growing recognition of the applicability of the biological machinery of photosynthesis as the active solar cell material. The primary advantage being that these molecular systems have been already optimized for light harvesting and charge separation by over 3 billion years of evolution. In theory the use of photosynthetic proteins may result in biophotovoltaic devices with open circuit voltages in excess of 1 V and non-limiting electron transfer rates1. The utilization of photosynthetic proteins as solar cell material may ultimately provide the cheapest possible photovoltaics devices since these key building blocks can be harvested from organisms such as algae in large scales. Biophotoelectrode designed for photovoltaics may also be applied for chemical fuel production2 and for novel sensing concepts.
To these ends, we design redox hydrogels for highly efficient electrical contacting and stabilization of the natural photosynthetic protein in artificial devices. While early devices yielded only low efficiency3, the fine tuning of electron mediators with redox potentials adjusted to the one of the protein resulted in several order of magnitude improvement in both photocurrents4 and voltages5. Together with our cooperation partners (W. Schuhmann, M. Nowaczyk and M. Rögner), we have shown that electrons provided by photosynthetic proteins can be recovered at artificial conducting materials, even faster than in natural photosynthesis itself4. The possibility to further improve these performances raise the expectation to eventually achieve practical efficiency from biophotovoltaic devices to provide electrical energy both in high tech devices, such as biocompatible photovoltaics in smart contact lenses6, and in low tech, “painted” large scale solar cells which opens new routes for the cost-per-Watt race in electrical energy production7.
- Plumeré, N. Nature Na notech. 2012, 7 (10), 616–617. DOI: 10.1038/nnano.2012.175
- Zhao F., Conzuelo F., Hartmann V., Li H., Nowaczyk M. M., Plumeré N., Rögner M., Schuhmann W., J. Phys. Chem. B, 2015, 119 (43), 13726–13731. DOI: 10.1021/acs.jpcb.5b03511
- Kothe, T.; Plumeré, N.; Badura, A.; Nowaczyk, M. M.; Guschin, D. A.; Rögner, M.; Schuhmann, W., Angew. Chem. Int. Ed. 2013, 52 (52), 14233–14236. DOI: 10.1002/anie.201303671
- Kothe T., S. Pöller, F. Zhao, P. Fortgang, M. Rögner, W. Schuhmann, N. Plumeré Chemistry – A European Journal, 2014, 20, 11029–11034. DOI: 10.1002/chem.201402585
- Hartmann V.,Kothe T., Pöller S., El-Mohsnawy E., Nowaczyk M. M., Plumeré N., Schuhmann W.,Rögner M., Phys. Chem. Chem. Phys., 2014, 16, 11936-11941. DOI: 10.1039/C4CP00380B
- Ravilious K, Clothes that recharge your laptop – the near-endless possibilities of artificial leaves. Horizon, The research & innovation magazine of the European commission. 16 October 2014
- Plumeré et al., Researchers forecast an Ebola vaccine and low-cost solar cells in 2015. Horizon, The research & innovation magazine of the European commission, 5 January 2015
The direct electron transfer between redox proteins and electrodes is a fundamental issue in bioelectrocatalysis, bioelectronics, biosensors, biofuelcells and nanobiotechnology. The goal of our research is to design functional surfaces on a molecular level to advance our ability to effectively control and direct the electrochemical activity of biomolecules.
We use organic chemistry, molecular biology and electrochemical approaches to build biologic-organic hybrid architectures. We are working on the development of nanosized chelators to achieve the specific recognition and orientation of a redox protein. Our interest is to gain fundamental information on the chelate effect on the supramolecular level. Understanding and adjusting the molecular recognition between the receptor and the guest in combination to designing electron relays to bridge redox enzymes are the key issues to achieve controlled orientation and fast electron transfer. We apply our redox protein immobilization concepts for sensing (nitrate biosensors) and energy conversion applications (bio-photovoltaics and bio-fuel cells).
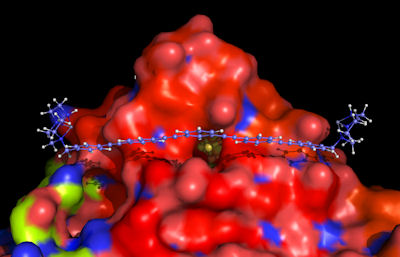